Andrea Morello, a prominent figure in quantum engineering, is paving the way for the future of quantum computing. As a Scientia Professor at UNSW and Program Manager for the ARC Centre of Excellence for Quantum Computation and Communication Technology, Morello’s work is pushing boundaries. His 2024 ARC Laureate Fellowship recognizes his critical role in the field, but the journey he describes is both complex and full of promise.
The Quantum Leap: Why Qubits Matter
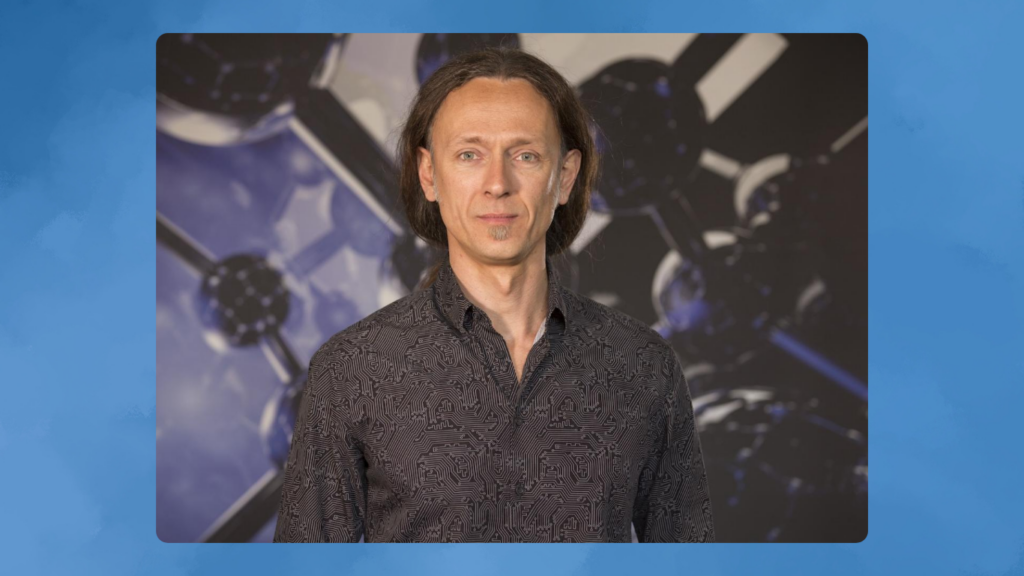
While classical computers rely on transistors to encode information in binary form—zeros and ones—quantum computing enters a new realm with quantum bits or qubits. These qubits aren’t limited to just zero or one. They can exist in a state of superposition, representing both simultaneously. Even more revolutionary is their ability to become entangled, meaning the state of one qubit can instantly affect another, regardless of distance. This opens the door to computations and problem-solving approaches that classical computers, no matter how advanced, simply cannot handle.
The fundamental advantage of quantum computing lies in this superposition and entanglement. With more qubits, the number of possible entangled states grows exponentially, enabling quantum computers to process vast amounts of data in parallel. For certain tasks—such as cryptography, data analysis, and drug discovery—quantum computing offers the promise of solving problems in minutes that might take classical computers thousands of years.
Reimagining Computing with Quantum Systems
But how does quantum computing actually work? In classical computers, transistors act as tiny switches, either open or closed, representing a 0 or a 1. These transistors are typically built into silicon chips, such as those found in mobile phones. Quantum computers, on the other hand, rely on qubits that encode information based on quantum properties. One method involves controlling an electron’s position, with left representing 0 and right representing 1. Another approach uses the spin of a particle, which is like a tiny magnetic needle pointing north or south, again corresponding to binary states.
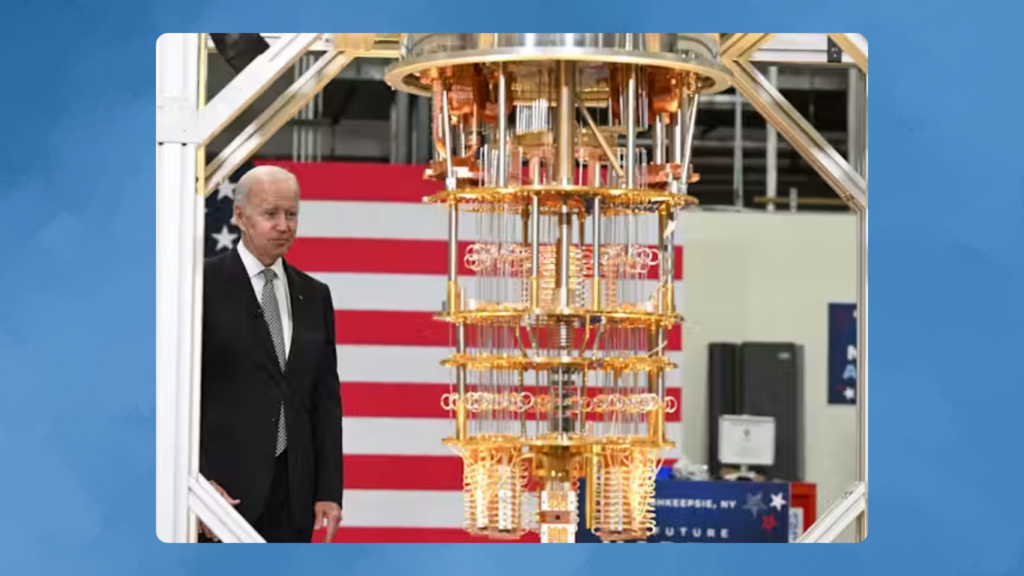
This raises a fundamental question: why use quantum systems when classical transistors perform the same function efficiently and cheaply? The answer lies in the quantum bit’s flexibility. While a classical bit can only be zero or one, a qubit can be both, allowing quantum computers to explore multiple possibilities simultaneously and solve problems more quickly.
Silicon: The Bedrock of Both Classical and Quantum Computers
The quest to build a quantum computer involves not just theorizing about qubits, but finding a practical way to control and manipulate them. For Morello and his team, the answer was silicon. This decision wasn’t arbitrary—silicon is the most extensively developed and reliable material in classical microelectronics. Over 60 years, the silicon industry has grown into a trillion-dollar powerhouse, producing chips with billions of perfectly functioning transistors. Leveraging this existing technology to build quantum computers offers a clear path to scalability and efficiency.
In fact, back in the 1990s, Bob Clark and Andrew Dzurak—pioneers in semiconductor microelectronics at UNSW—began exploring the possibility of capturing and controlling single electrons in silicon. While initial attempts failed, they laid the groundwork for future breakthroughs. It wasn’t until 2006, when Morello joined the project, that things began to take off.
A Breakthrough with Phosphorus Atoms
Morello’s contribution, alongside Andrew Dzurak and David Jamieson, came in the form of embedding a single phosphorus atom inside a silicon chip. Phosphorus atoms are already widely used in classical transistors to adjust their electrical properties, but in this case, the goal was different: to isolate one atom. This atom could then hold two qubits—one in its electron spin and the other in its nuclear spin.
In 2010, the team published a paper in Nature showing they could observe and control the spin of a single electron in real-time. This milestone demonstrated that silicon could indeed support quantum computing. The breakthrough opened new possibilities for scaling quantum computers while using silicon’s well-established infrastructure.
Challenges Ahead: Scaling Up to Industrial Quantum Computing
Despite these breakthroughs, the next step—building large-scale, commercially viable quantum computers—poses a significant challenge. Morello is clear: the cost of developing quantum computers that can tackle real-world problems will reach billions of dollars. While academic research can provide the foundations, the transition from lab to large-scale production will require a collaboration between researchers, industry leaders, and governments.
Morello emphasizes that his role as a professor is to develop new ideas, push the boundaries of what’s possible, and demonstrate that quantum computing can be realized on a silicon platform. The hope is that industry will pick up these ideas and turn them into scalable, commercial solutions. This is why focusing on silicon is so important—by working with a material that’s already integral to the tech industry, the leap from theory to application can be more seamless.
A Glimpse into the Future: What Quantum Computers Can Achieve
The potential applications of quantum computers are staggering. From revolutionizing cryptography to accelerating the discovery of new drugs, quantum computers will reshape entire industries. Their ability to process massive datasets and explore multiple possibilities at once means they could solve problems that are currently beyond the reach of even the most advanced classical computers.
However, the road to fully functional quantum computers is still long and filled with technical and financial hurdles. Morello believes that by leveraging the world’s existing silicon infrastructure, quantum computing can advance more rapidly. The goal is not just to build quantum computers, but to ensure they can solve real-world problems more efficiently and faster than ever before.
In short, quantum computing is on the brink of transforming the future, but the path ahead will require continued breakthroughs, significant investment, and collaboration across industries. By building on the existing foundation of silicon technology, the transition to quantum computing could happen sooner than expected.
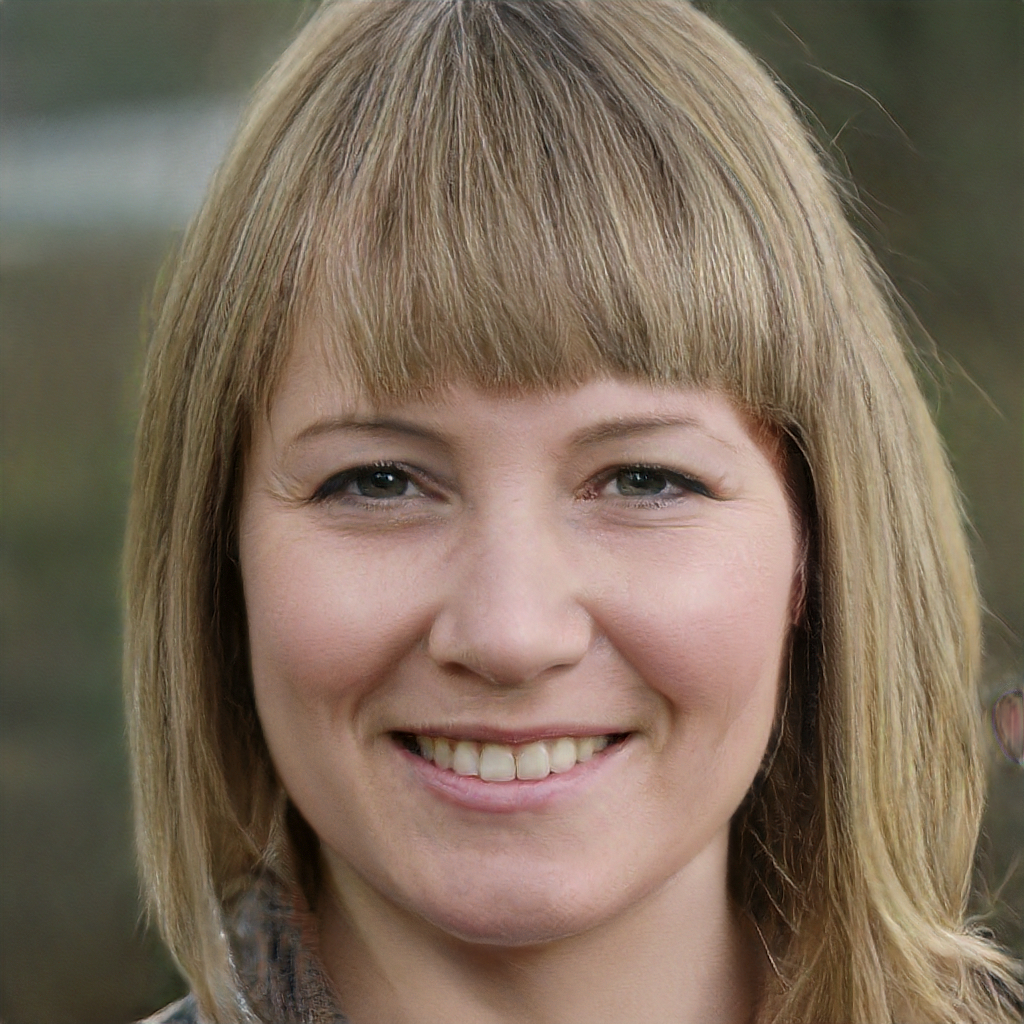
Subtly charming pop culture geek. Amateur analyst. Freelance tv buff. Coffee lover