Researchers at ETH Zurich have demonstrated a groundbreaking method for controlling the quantum states of single electron spins using currents of electrons with aligned spins. This technique, which promises future applications in electronic circuit elements, marks a significant advancement in the field of quantum computing.
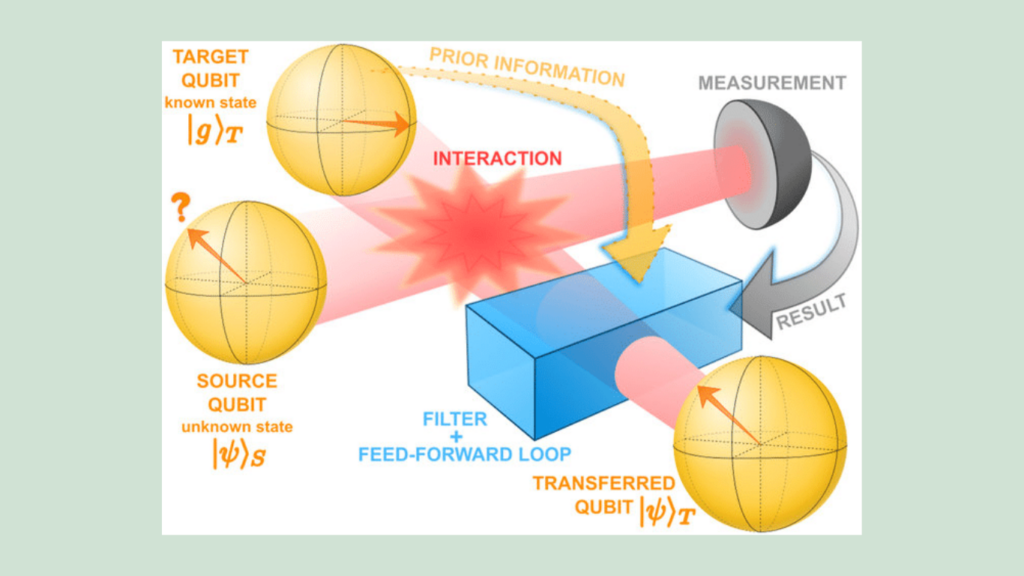
Spintronics: A New Frontier for Data Storage and Processing
Electron spins, integral to both classical and quantum data storage, are now being harnessed for more advanced applications. Traditionally, single electron spins are manipulated using electromagnetic fields. However, the ETH Zurich team has shown that spin-polarized currents—currents of electrons whose spins are uniformly aligned—can selectively manipulate these quantum states. This innovative approach could precisely control quantum states in magnetic qubits, essential for the development of quantum devices.
The Dual Nature of Electrons
Electrons possess an intrinsic angular momentum known as spin, allowing them to align along magnetic fields similar to compass needles. Beyond their electric charge, which dictates their behavior in electronic circuits, the spin of electrons is increasingly utilized for data storage and processing. Current technologies like Magnetic Random Access Memory (MRAM) already leverage spin-polarized currents to alter magnetization at specific material points. This existing technology underscores the potential of spin-polarized currents in controlling quantum states.
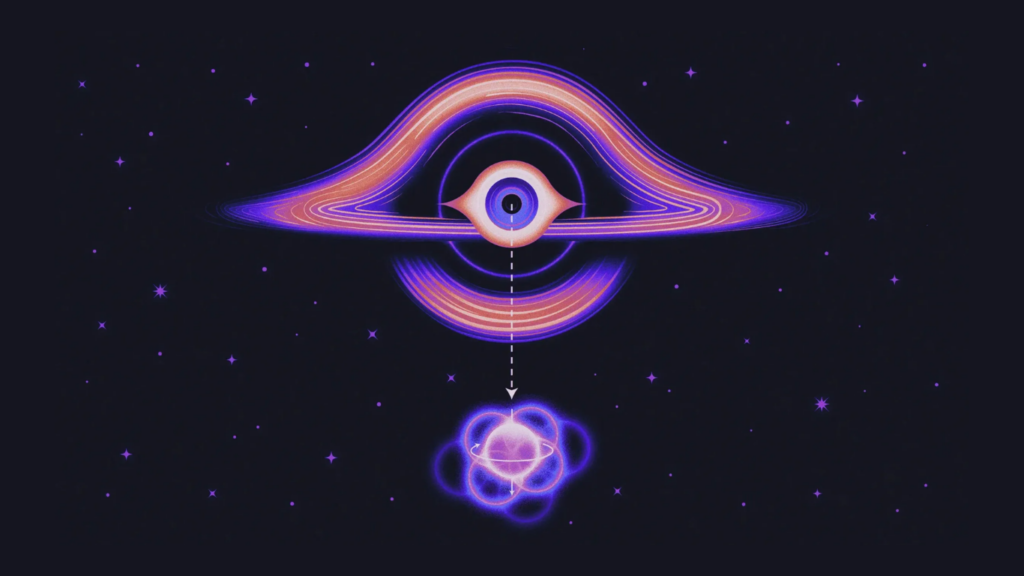
Breakthrough at the Quantum Level
Pietro Gambardella and his team at ETH Zurich have published their findings in the journal Science, demonstrating that spin-polarized currents can indeed control the quantum states of single electron spins. This discovery could revolutionize how quantum states are manipulated in various technologies, particularly in quantum computing.
The Experiment: Tunnel Currents and Quantum States
To delve deeper into the quantum mechanical processes, the researchers prepared pentacene molecules on a silver substrate with a thin insulating layer of magnesium oxide. This setup allowed electrons in the molecule to behave similarly to those in free space. Using a scanning tunneling microscope, the team characterized the electron clouds in the molecule by measuring the tunnel current—the current created when electrons tunnel quantum mechanically from a tungsten needle tip to the molecule.
Creating a Miniature Magnet
By picking up a few iron atoms with the tungsten tip, the researchers created a miniature magnet. When a tunnel current flowed through this magnet, the spins of the electrons aligned parallel to its magnetization. By applying a constant voltage along with a fast-oscillating voltage to the magnetized tip, they measured the resulting tunnel current. The researchers observed characteristic resonances in the tunnel current, varying the strength and frequency of the voltages to draw conclusions about the processes between the tunneling electrons and the molecule.
Insights and Implications
The data revealed two key insights. First, the electron spins in the pentacene molecule responded to the electromagnetic field created by the alternating voltage as they would in traditional electron paramagnetic resonance. Second, the shape of the resonances suggested an additional process influencing the electron spins in the molecule. This process, known as spin transfer torque, changes the molecule’s spin under the influence of a spin-polarized current without direct electromagnetic field action. The ETH team demonstrated that this method could create quantum mechanical superposition states of molecular spins, crucial for quantum technologies.
Future Applications and Precision Control
Spin control by spin-polarized currents at the quantum level offers various potential applications. Unlike electromagnetic fields, spin-polarized currents act very locally and can be steered with precision to less than a nanometer. This precision could enable extremely accurate addressing of electronic circuit elements in quantum devices, controlling the quantum states of magnetic qubits and advancing quantum technology.
This innovative method by ETH Zurich researchers paves the way for new applications in quantum computing and spintronics, potentially transforming how we store, process, and manipulate data at the quantum level.
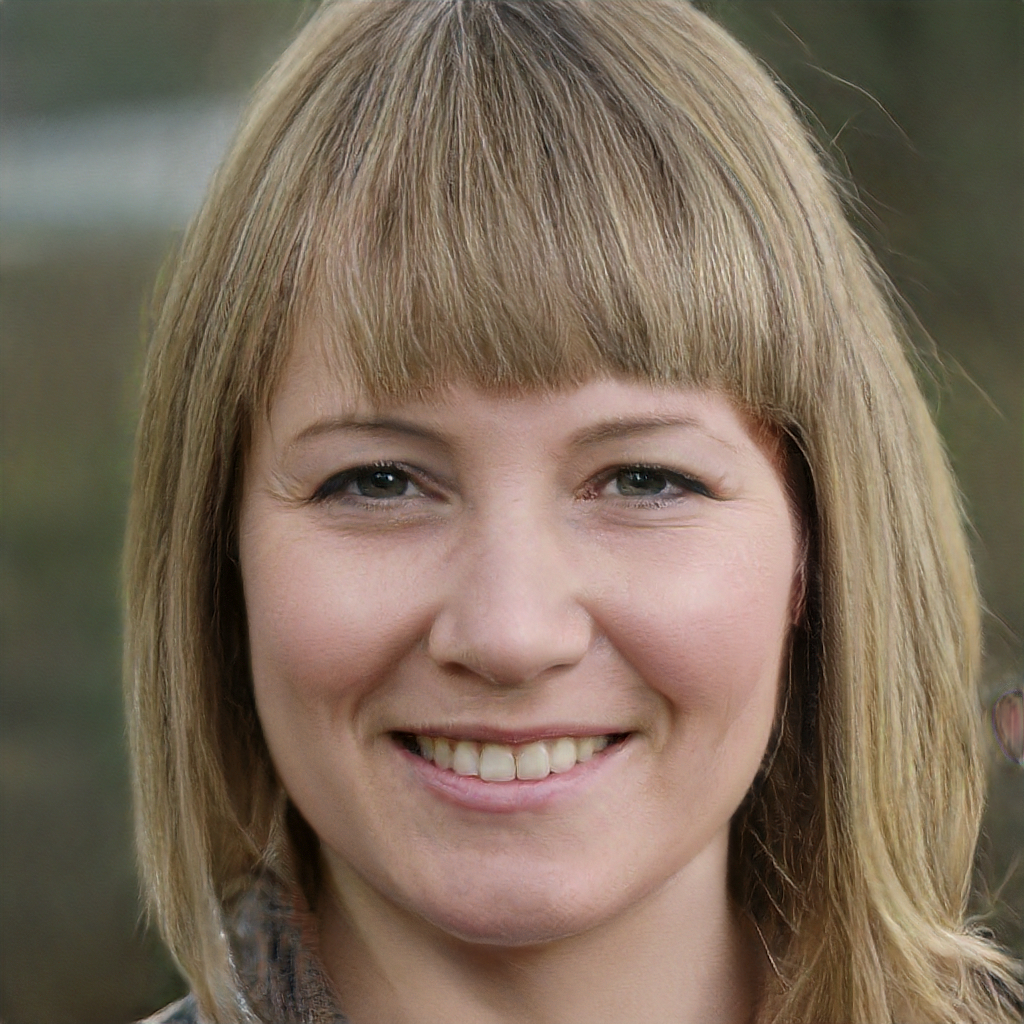
Subtly charming pop culture geek. Amateur analyst. Freelance tv buff. Coffee lover